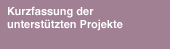

|
 |
 |
de | fr | en Druckansicht 
3R-Project 78-01
Validation of a combined perfusion/loading chamber for ex-vivo bone metabolic studies and bone-biomaterial interactionsR. Geoff Richards AO Research Institute, 7270 Davos, Switzerland geoff.richards@aofoundation.org Keywords: sheep; bone, cartilage; perfusion chamber; reduction; replacement; validation Duration: 3 years Project Completion: 2005 Background and Aim To date most in vitro work on trabecular bone is performed with either cell monolayers or organ cultures. However, both of these techniques have limitations: cell monolayers lack some types of bone and marrow cells as well as the native three dimensional structure of bone, while organ cultures can only be sustained for 3-5 days before necrosis sets in, due to oxygen diffusion limitations. Numerous studies are also carried out in vivo especially using sheep models. These also have their limitations, including both ethics & costs. To overcome these drawbacks, an ex vivo system for the long term culture of trabecular bone, was developed, “ZETOS”, (D. Jones, Marburg, Germany and E. Smith, Madison, Wisconsin, 1997). ZETOS maintains the three dimensional structures of trabecular bone, and can keep all cell types (osteoblasts, osteoclasts, osteocytes and marrow cells) viable for at least 30 days (1). ZETOS has a unique loading system that allows physiological levels of strain in bone samples to be measured online. With this model, the process of bone ingrowth onto biomaterial surfaces can be studied ex vivo. The aims of the project are 1) To calibrate the loading system, so that the displacement measured on the loading device can be correlated to the actual displacement that the bone receives. 2) To initiate the validation of the ZETOS system for ovine, bovine and human bone samples; Most in vivo tests for orthopaedics are carried out in sheep and this tissue can be used for comparison purposes. Since the system can use human bone it has the advantage of not being dependent on any shortcomings of animal models of osteoporosis and can use real human osteoporotic bone. Two advantages of bovine tissue are that firstly many antibodies are available against bovine proteins and secondly it is much easier (at least in Davos) to attain young bovine samples compared to sheep and human tissue.
Method and Results Bone growth ex vivo: sheep femora, bovine metacarpals or human femoral heads were cut at the joint into 7 mm thick sections. Cancellous bone cores, 10 mm in diameter, were drilled from the sections and then cut parallel to the height of 5 mm. Throughout all cutting procedures the bone was irrigated with sterile saline to limit the amount of damage caused by heat and to stop the bone from drying out. Bone cores were washed in cell culture medium (containing tested foetal calf serum) and antibiotics for 30 minutes before being inserted into the chambers. The assembled system was allowed to settle for 36 hours at 37°C (Fig. 1). The set up for the Zetos system consists of two parts. One part is a number of cross-flow circumfusion chambers, where trabecular bone cores are situated. These cores are circumfused by a constant flow of media, which is circulated in a closed system for 1-2 days. The second part is a computer controlled loading device, which is used to maintain the bone in a loaded environment resembling that of the in vivo situation. Without load, bone is believed to have a net increase in resorption, as is seen in patients after prolonged bed rest or astronauts in zero gravity. Inside the loading device there is a piezo stack that expands and contracts under high voltage, placing a force on the piston of the chambers. This force in turn causes a deformation (compression) of the bone cores. The expansion/contraction of the piezo is controlled by a sensor that acts in a feedback loop to control the amount of force applied to each chamber, thus in turn controlling the amount of deformation the bone cores receives. For 5 minutes daily, each chamber, with the bone core inside, is placed inside the loading device. The computer is programmed so that the piezo expands and contracts to give the bone compression of 20 and 5 micrometer in a physiological (walking, jumping) waveform at 1 Hz (once a second) for 300 cycles (5 min). By measuring the force required to deform the bone cores it is possible to calculate the apparent Young’s modulus of each sample. This is a measure of its apparent stiffness, which is given in the unit Pascal. Previous work in Marburg showed that the chamber apparently maintained both the three dimensional structure of the bone as well as cell viability. Our hypothesis is that loaded bones should become stiffer, with a net increase of bone formation, due to the mechanical stimulation. Conversely, unloaded bone should reduce its stiffness, due to either a net increase in bone resorption and/or a net reduction in bone formation. Achievements To date we have cultures all three models (ovine, bovine and human) in the Zetos system. Several cell types have been viewed including, osteoblasts osteoclasts, osteocytes, lining cells, adipocytes, blood vessels, and haemopoietic cells (Figure 2). The three models have been investigated with regard to general histolomorphology, bone density with the use of radiographs, bone 3D structure with microcomputer tomography (mCT) and scanning electron microscopy (SEM) imaging (Figure 3). Molecular and histological techniques have been investigated to demonstrate cell viability and protein synthesis. The viability stain Cell Tracker Green and Ethidium homodimer 1 have been seen to be retained within cells through the fixation, dehydration and embedding procedure. This can therefore be applied in future Zetos experiments to demonstrate percentage of viable cells as the Cell Tracker Green enters viable cells and is cleaved by esterases in the cytoplasm and fluoresces in green, the ethidium homodimer binds to DNA of non viable cells and fluoresces in red. Immunohistochemistry techniques have also been developed to allow localisation of bone marker proteins such as alkaline phosphatase, osteonectin and RANKL. We are currently working on localising osteocalcin, osteopontin and procollagen type I for light microscopy and electron microscopy (Figure 4 & 5). These methods of viewing the bone specific proteins will be applied to bone core samples that have been cultured within the Zetos system for the validation of the system. The Zetos loading has now been fully calibrated so that the displacement measured on the loading device can be correlated to the displacement that the bone receives. This was carried out with the aid of our collaborators in Marburg. A number of computer macros have been programmed for the Zeiss Axioplan imaging system allowing the number of cells, thickness of osteoid seems, bone apposition rate to be calculated.
Conclusions and Relevance for 3R In 2003 we hope to show that the three-dimensional structure and viability of the ovine, bovine and human bone grown in this ex vivo system is comparable to that of the in vivo situation. We also intend to show that we have on-line protein production in the cells by using the above-mentioned immunohistological staining, and 3H-glycine incorporation as markers. After completion of the validation we would hope to study the effect of load and various chemical stimulators to force bone growth and force resorption. Other future work will be to evaluate standard biomaterial surfaces with this system and compare the results to the in vivo situation. This will all aid the goal of reducing the number of animals used in experiments to evaluate biomaterials and their surface coatings. By using human tissue we may be able to eliminate the need for an animal model to study osteoporosis in cancellous bone. In addition, the system may be useful for studying bone metabolism in pharmaceutical research and development, which would again reduce the numbers of animal experiments. (see also 3R-Info-Bulletin Nr. 28) Published updated Version 28/2007 (pdf) References 1. E. L. Smith, F. Martens, K. Koller, W. Clark, D. B. Jones. The Effects of 20 Days of Mechanical Loading Plus PTH on The E-Modulus of Cow Trabecular Bone, Abstracts 22nd annual meeting ASMBR 2000 SAP 07 2. D.B.Jones, U.Boudriot, M.Kratz, F. Martens, K. Koller, E. L. Smith (2001) A Trabecular Bone and Marrow Bioreactor. European Cells and Materials Vol. 1. Suppl. 2, 2001 (page 53) 3. C.M.Davies, D.B.Jones, R.G.Richards (2001) Trabecular Bone and Marrow Bioreactor. Preliminary Results of Culturing and Loading 3D Cores of Sheep Bone (Ex vivo System). European Cells and Materials Vol. 1. Suppl. 2, 2001 (page 71-73) 4. C.M. Davies, D.B. Jones, M. Alini, C. Archer, R.G. Richards (2001) Ex-vivo trabecular bone percolation system: development for evaluation of implant surfaces. European Cells and Materials Vol. 2. Suppl. 1, 2001 (page 63). 5) Stoddart MJ, Furlong PI, Simpson A, Davies CM, Richards RG (2006) A comparison of non-radioactive methods for assessing viability in ex vivo cultured cancellous bone. Eur Cell Mater 12, 16-25. can be downloaded for free from http://www.ecmjournal.org/journal/papers/vol011/vol011a07.php 6) Davies CM, Jones DB, Stoddart MJ, Koller K, Smith E, Archer CW, Richards RG (2006) Mechanically loaded ex vivo bone culture system ‘Zetos’: Systems and culture preparation. Eur Cell Mater 11 57-75. can be downloaded for free from http://www.ecmjournal.org/journal/papers/vol012/vol012a02.php The project is carried out in collaboration with: Prof. D.B. Jones Experimental Orthopaedics & Biomechanics, Philipps University Marburg Germany. jones@post.med.uni-marburg.de Prof. C. Archer Cardiff School of Biosciences, Cardiff University, Wales (GB) archer@cardiff.ac.uk
Figures 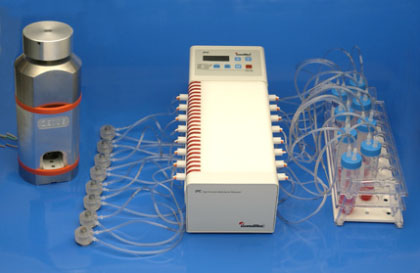 Figure 1: System set up in the 37° C room once the bone preparation was complete. Media was circumfused through the bone chamber and bone core without loss of nutrients or the excess build up of waste, with media changed every 2 days.
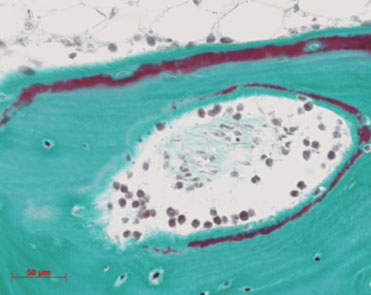 Figure 2: Histiological image of human bone cultured in the Zetos system for 30 days, stained with Masson Goldner stain, which shows the mineralised bone as green, the osteoid and cells in red.
Figure 3 Scanning electron micrographs of ovine bovine and human tissue, respectively, to compare the difference in bone structure and density. Figure 4 Histiological image of human bone labelled with a primary antibody for alkaline phosphatase.
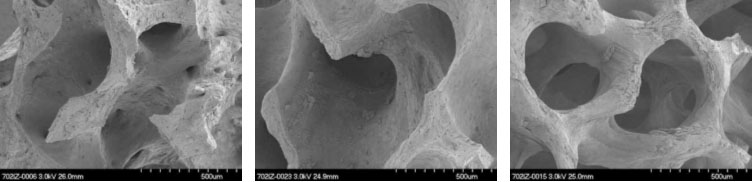 Figure 5: Scanning electron micrographs of a human bone sectioned labelled with a primary antibody directed against RANKL.
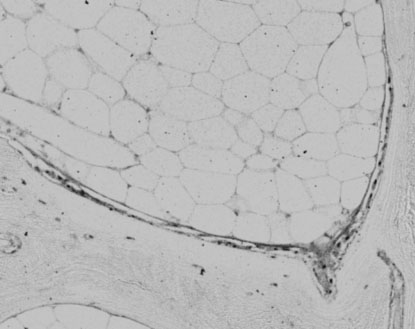 Figure 4
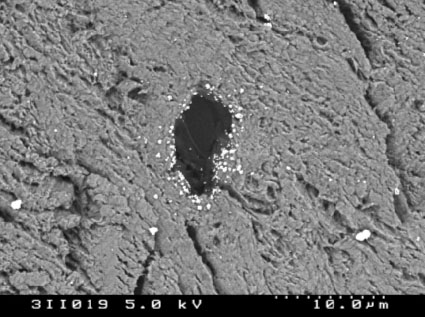 Figure 5
|